The Brilliant Green Complete Single Collection 7-8 Rar
Posted By admin On 19/04/19~Release by the brilliant green(see all versions of this release, 5 available)
- Angel song –イヴの鐘– (backing track) by the brilliant green (track 11) そのスピードで (backing track) by the brilliant green (track 5) 冷たい花 (backing track) by the brilliant green (track 4).
- The Brilliant Green Complete Single Collection 97 08 Rar Download The Brilliant Green Complete Single Collection 97 08 Rarity Furk.net is not a file locker and doesn't support filesharing for profit.
Complete single collection ’97–’08 ~ Release by the brilliant green.
Tracklist
▼ CD 1 | |||
---|---|---|---|
# | Title | Rating | Length |
1 | Bye Bye Mr. Mug | 4:33 | |
2 | goodbye and good luck
| 4:33 | |
3 | There will be love there –Ai no Aru Basho–
| 4:01 | |
4 | Tsumetai Hana
| 4:37 | |
5 | Sono Speed de
| 5:11 | |
6 | Nagai Tameiki no You ni
| 5:42 | |
7 | Ai no♥Ai no Hoshi
| 3:52 | |
8 | CALL MY NAME (Japanese version) | 5:14 | |
9 | BYE! MY BOY!
| 3:25 | |
10 | Hello Another Way –Sorezore no Basho–
| 5:35 | |
11 | angel song –Eve no Kane–
| 4:24 | |
12 | Forever to me ~Owari Naki Kanashimi~
| 5:04 | |
13 | Rainy days never stays
| 3:55 | |
14 | I’M SO SORRY BABY
| 4:49 | |
15 | Stand by me
| 3:41 | |
16 | Enemy
| 4:58 | |
17 | Ash Like Snow
| 5 | 4:32 |
Credits
CD 1
arranger: | the brilliant green (tracks 3–7, 9, 11, 17) |
---|---|
composer: | |
lyricist: | 川瀬智子 (tracks 3–7, 9–14, 17) |
publisher: | エムシーキャビン音楽出版 (tracks 3, 5) フジパシフィック音楽出版 (– 2014-12-31) (track 5) |
karaoke versions: | Ash Like Snow (original instrumental) by the brilliant green (track 17) BYE! MY BOY! (backing track) by the brilliant green (track 9) Enemy (original instrumental) by the brilliant green (track 16) I’M SO SORRY BABY (backing track) by the brilliant green (track 14) Stand by me (original instrumental) by the brilliant green (track 15) angel song –イヴの鐘– (backing track) by the brilliant green (track 11) そのスピードで (backing track) by the brilliant green (track 5) 冷たい花 (backing track) by the brilliant green (track 4) 長いため息のように (backing track) by the brilliant green (track 6) |
later translated versions: | |
later versions: | goodbye and good luck (piano arrange version) (track 2) |
recording of: | Ash Like Snow(機動戦士ガンダム00) (track 17) Bye Bye Mr.Mug (track 1) Enemy (track 16) Hello Another Way –それぞれの場所– (track 10) Rainy days never stays (track 13) There will be love there –愛のある場所– (track 3) goodbye and good luck (track 2) 冷たい花 (track 4) 長いため息のように (track 6) |
Release
transliterated/translated track listing of: | complete single collection ’97–’08 |
---|
Release Group
other databases: | https://www.generasia.com/wiki/the_brilliant_green_Complete_Single_Collection_'97-'08[info] |
---|
Abstract
All-trans-retinoic acid (atRA) is an essential signaling molecule in embryonic development. It regulates cell differentiation by activating nuclear retinoic acid receptors (RAR) and retinoid-X receptors (RXR), which both control gene expression. In addition, atRA could act in the cytoplasm by modulating the activity of mitogen-activated protein kinases (MAPK) ERK and p38, which also have a role in cell differentiation. AtRA can induce the differentiation of P19 embryonic carcinoma stem cells into adipocytes, cardiomyocytes, and skeletal muscle cells, concurrently, in the same culture. We postulated that combinations of atRA, atRA analogs exhibiting selectivity for RAR or RXR, and inhibitors of ERK and p38 signaling (ERKi and p38i) could be used to favor one mesodermal fate over the others in the P19 model. In a first series of experiments, we replaced atRA by an agonist of RXR (LG100268) or RAR (TTNPB) to preferentially stimulate one group of receptors over the other. LG100268 was as adipogenic and myogenic as atRA, whereas TTNPB strongly induced adipogenesis, but not myogenesis. ERKi enhanced the myogenic action of atRA, and p38i increased both adipogenesis and myogenesis. In a second series of experiments, we combined atRA with an RAR or RXR antagonist (RARatg or RXRatg) to preferentially deactivate each receptor group in turn. The combinations atRA+RXRatg and atRA+RARatg, including or not ERKi, had similar mesodermal actions as atRA. In contrast, there was no myogenesis with atRA+RXRatg+p38i treatment, and there were no myogenesis and no adipogenesis with the atRA+RARatg+p38i combination. Overall, the results indicate that p38 has a role in mesodermal differentiation that depends on the retinoid context. Indeed, p38 in conjunction with RXR is important in myogenesis, and p38 and RAR in adipogenesis. Under the conditions tested, it was possible to stimulate adipogenesis with a block on myogenesis, whereas increased myogenesis was accompanied by adipogenesis.
Introduction
All-trans-retinoic acid (atRA), the active derivative of vitamin A, is a signaling molecule that is essential to the proper development of the vertebrates [1,2]. It regulates cell proliferation and differentiation by activating two groups of nuclear receptors, the retinoic acid receptors (RAR) and the retinoid-X receptors (RXR) [1]. Both receptor groups comprise α-, β-, and γ-isoforms that associate into dimers within the same group (RAR:RAR) or between the groups (RAR:RXR) [1,3]. These dimers are the functional DNA-binding units that control expression of many genes, including transcription factors [1,3]. RAR and RXR have distinct binding properties, which have been revealed with 9-cis-RA, a naturally occurring isomer of atRA. In fact, in vitro, RAR can bind both atRA and 9-cis-RA, whereas RXR bind only 9-cis-RA [4]. In vivo, atRA, which does not bind itself to RXR, can however modulate the activity of these receptors through isomerization into a form recognized by them or through the formation of the RAR; RXR dimers [5,6]. Synthetic retinoids having a binding specificity for RAR or RXR can provide molecular tools to distinguish RAR and RXR influence in the effects of atRA on cell proliferation and differentiation. Conversely, retinoids could be also used to control these cellular functions. Various synthetic RAR and RXR ligands have indeed been developed to reduce cell proliferation in cancer [7,8], but they have been rarely exploited to direct differentiation of embryonic and stromal stem cells.
AtRA can trigger the differentiation of embryonic stem (ES) cells and embryonic carcinoma (EC) cells in culture. Its action varies in concentration- and time-dependent manners [9]. For instance, if mouse P19 EC cells are exposed to 10−6 M atRA when they begin to form embryoid bodies in culture, they differentiate into neurons [10,11]. In contrast, when atRA is added 2 days later, P19 cells undergo mesodermal differentiation, leading to the concurrent generation of adipocytes, cardiac muscle (CM) cells, and skeletal muscle (SKM) cells in the same culture [12]. With the use of this unique differentiation protocol and synthetic retinoids, P19 cells thus provide a model to study the influence of RAR and RXR in the generation of multiple mesodermal cell derivatives. Partial information on retinoid influence has been obtained from ES cell lines or from P19 cells, but by evaluating each cell fate independently, under conditions those generated adipocytes and no myocytes, or myocytes and no adipocytes. Specifically, RXR agonists were shown to increase differentiation into muscle cells [13,14], while a RARβ-specific agonist was found to trigger adipogenesis [15].
Like atRA, mitogen-activated protein kinases (MAPK) play an essential role in cell proliferation and differentiation [16–20]. These kinases are components of signaling cascades that relay extracellular stimuli to transcription factors in the nucleus. MAPK are activated/phosphorylated or inactivated/dephosphorylated depending on the stimuli. MAPK ERK and p38 have been implicated in mesodermal differentiation, but information is not complete or clear. P38 has an antiadipogenic action [21], while its activity appears to be required in myogenesis [17,22–26]. However, three recent studies have shown that the use of small concentrations of the p38 inhibitor SB203580 (≤10μM) rather promotes than inhibits cardiomyogenesis [27–29]. ERK activity is required for adipogenesis [30–33], but its influence in myogenesis is uncertain. Indeed, ERK was either judged to be unnecessary or necessary to myogenic differentiation [20,23,24]. Overall, those studies relied on different cell differentiation protocols to induce adipogenesis and myogenesis, preventing the unambiguous determination of the extent to which ERK and p38 influence undifferentiated cells to become adipocytes or myocytes.
There could exist different signaling relationships between atRA and MAPK in cell differentiation. MAPK activity can be modulated by various stimuli in absence of atRA, but it can also be modulated as a consequence of changes in gene expression induced by the action of atRA on nuclear RAR/RXR. In addition, MAPK can be activated or inactivated by atRA through a mechanism that is independent of RAR-/RXR-mediated gene transcription [34,35]. This nongenomic mechanism of atRA action involves RAR pools present in the cytosol or at the plasma membrane, and the incorporation of these RAR into signaling complexes that modulate MAPK activity [36]. Activated MAPK phosphorylate various targets, including RAR and RXR, themselves and their coregulators, and thus fine tune positively or negatively the transcriptional activity of these receptors. Activation of MAPK by RAR can occur within minutes or hours, and be reverted rapidly or last a long time [34,37,38]. Pharmacological inhibition or gene silencing of MAPK has been used to sensitize cancer cells to the antiproliferative or prodifferentiating action of atRA and retinoids [39,40]. This strategy could be used to direct stem cell differentiation toward a particular lineage.
The collection of studies suggested us that modulation of RAR and RXR signaling in conjunction with inhibition of ERK or p38 signaling could direct cell differentiation into adipogenic or myogenic routes. To test this hypothesis, we used P19 cells, whose adipogenic and myogenic routes are activated concurrently in response to atRA. We compared the effect of atRA on cell proliferation and differentiation with that of treatments that preferentially stimulate RAR or RXR (by using an RAR- and RXR-specific agonist) or preferentially deactivate RAR or RXR (by using atRA in combination with an RAR- or RXR-specific antagonist). The impact of introducing the inhibitors of ERK and p38 signaling in these treatments was also evaluated. Our results provide a better appraisal of RAR and RXR influence in the effects of atRA on stem cells, evoking the possibility of using the RAR and RXR ligands to direct cell differentiation in culture. Ultimately, such information could help in the development of cell-based therapies in regenerative medicine.
Materials and Methods
Retinoids and MAPK modulators
AtRA and TTNPB (that is, E-4-[2-(5,6,7,8-tetrahydro-5,5,8,8-tetramethyl-2-naphthylenyl)-1-propenyl] benzoic acid) were from Sigma-Aldrich. LG100268 (LG268), LG100815, and LG101208 were generously given by Ligand Pharmaceuticals. LG100815 and LG101208 are, respectively, referred to as an RAR antagonist (RARatg) and RXR antagonist (RXRatg) throughout. Inhibitors of ERK and p38 signaling, respectively, PD98059 and SB203580, were from Cedarlane Labs. Data warehousing data mining and olap alex berson pdf.
Culture and treatment of P19-MLC2v-GFP cells
The MLC2v-GFP clone of the mouse P19 cell line was used in all experiments. This clone carries the green fluorescent protein (GFP) gene under the transcriptional control of the promoter of ventricular myosin light-chain-2 (MLC2v) [41]. Cells were routinely cultured at 37°C, in a humidified atmosphere of 5% CO2, and in a propagation medium consisting of alpha-modified Eagle's minimum essential medium (αMEM; Wisent) supplemented with 10% heat-inactivated fetal bovine serum (PAA Laboratories), 50U/mL penicillin, and 50μg/mL streptomycin (HyClone Laboratories) [12,42]. The cells were passaged every 2 days using trypsin and EDTA. Cells were kept under G418 selection (400μg/mL; HyClone Laboratories) until proliferation and differentiation experiments.
For proliferation studies, cells were seeded in tissue culture-grade plates and grown, for 48h, in a propagation medium supplemented with 10−6 M retinoid, and then analyzed by Crystal Violet staining.
For mesodermal differentiation, cells were manipulated as described by Bouchard and Paquin [12] and schematized in Fig. 1A. Briefly, cells were grown, from day zero (D0) to D2, in hanging drops of 20μL of the propagation medium containing 1000 cells, to initiate aggregation. The cell aggregates (embryoid bodies) were transferred into bacteriological grade-plates and cultured in suspension for 3 days, in a propagation medium supplemented with 10−6 M atRA. This medium was renewed every day (from D2 to D5). Aggregates were maintained in suspension for another 2 days in absence of atRA (D5 to D7). Afterward, aggregates were transferred into tissue culture grade-plates and grown as adherent cultures for the following 20 days (D7+20). The adherent cultures were maintained in a maturation medium consisting of a propagation medium supplemented with 85nM insulin and 2nM triiodothyronine (T3; Sigma-Aldrich). This medium was renewed every second day. To study retinoid-specific effects, TTNPB, LG268, RARatg, and RXRatg (10−6 M each) were used in the place of, or in combination with, atRA. In some studies, PD98059 or SB203580 (10μM each) was combined with atRA and retinoids; in these cases, these inhibitors were added 15min before atRA and retinoids. Noninduced (NI) cultures were subjected to the aggregation and postaggregation procedures, but in the absence of inducing agents. At the end of differentiation, cells were analyzed by PCR, Oil-Red-O staining, cytofluorescence, and immunoblotting.
Overview of cell treatments. (A) For mesodermal differentiation, P19-MLC2v-GFP cells were grown as suspended aggregates during the induction phase (D0 to D7) and as adherent cultures during the maturation phase (from D7 forward). The inducing agent was all-trans-retinoic acid (atRA) provided to the culture medium from D2 to D5. Modifications of this protocol included the replacement of atRA by another retinoid, the use of atRA in combination with another retinoid, and the combination of atRA or atRA+retinoid with mitogen-activated protein kinase (MAPK) inhibitors PD98059 and SB203580. (B) Structure of retinoids. AtRA and 9-cis-RA are physiologically relevant prototype ligands of retinoic acid receptors (RAR) and retinoid-X receptors (RXR), respectively. TTNPB and LG100815 (RARatg) are, respectively, synthetic pan-agonist and pan-antagonist of RAR. LG100268 (LG268) and LG101208 (RXRatg) are, respectively, synthetic pan-agonist and pan-antagonist of RXR.
Crystal violet staining
Cells were fixed for 20min in phosphate buffer saline (PBS) containing 4% para-formaldehyde, rinsed in PBS, and covered for 30min with PBS containing 0.1% Crystal Violet (Sigma-Aldrich). The dye retained by cells was extracted with 10% acetic acid and measured by absorbance at 595nm [12].
Polymerase chain reaction
Total RNA was extracted with Trizol Reagent (Invitrogen), UV-quantified, and diluted in diethylpyrocarbonate treated-water to a concentration of 0.1μg/μL. Any DNA in the extracts was digested by incubation with recombinant DNase 1 (Roche Diagnostics) for 15min at 37°C. Integrity of total RNA and absence of DNA contamination in extracts were verified by electrophoresis in 1% agarose gels. Reverse transcription (RT) was done using an Omniscript Reverse Transcriptase kit (Qiagen) and oligo-dT (Amersham Biosciences). Reactions ran 1h at 37°C, followed by 5-min inactivation at 95°C. Quantitative real-time PCR (qPCR) amplifications were done with a LightCycler instrument (Roche Diagnostics) using a QuantiTect SYBR Green PCR Kit (Qiagen), the cDNA, and 0.5μM of combined forward and reverse specific primers (Invitrogen). Primer sequences and qPCR conditions are detailed in Supplementary Table S1 (Supplementary Data are available online at www.liebertpub.com/scd). Fifty cycles enabled the development of the exponential amplification phase, and data were quantified using the Ct approach (fluorescence threshold) and the RelQuant software (Roche Diagnostics). Data were normalized with respect to GAPDH or actin expression as an endogenous RNA standard. Size and purity of the qPCR products were verified on agarose gels. Absence of DNA contamination was verified by amplifying the total RNA samples not subjected to RT.
Oil-Red-O staining
Cell cultures were fixed for 20min in PBS containing 4% para-formaldehyde, rinsed in PBS, covered for 3min with 100% propylene glycol and then for 60min with 0.5% w/v Oil-Red-O (Sigma-Aldrich) in 60% isopropanol, rinsed for 1min with 60% propylene glycol, and dried at 37°C for 60min. Dye retained by cells was extracted with isopropanol and measured by absorbance at 510nm. Cultures were then counterstained with Crystal Violet to correct the Oil-Red-O values for variations in cell culture densities. Oil-Red-O staining was also evaluated on micrographs [43]. In this case, after staining with Oil-Red-O, the cultures were covered with PBS and photographed with a Scion 12-bit Color FireWire 1394 Camera (Scion Corporation) mounted on a Nikon Eclipse Ti microscope. A transparent grid comprising 480 squares was placed on the micrographs to count the squares containing the stained cells. The results are expressed as percentages of the total number of squares (% surface occupancy). At least, three fields were photographed for each dish and averaged to represent the dish status. The fields of complete cellular confluency were chosen for all dishes.
Cytofluorescence
Cell cultures were fixed for 20min in PBS containing 4% para-formaldehyde, rinsed in PBS, and stored at 4°C in this buffer until analysis. Cells were permeabilized for 10min in PBS containing 1% Triton X-100, blocked for 60min in PBS containing 1.5% horse serum, and successively incubated for 60min each time, with mouse anti-sarcomeric (skeletal and cardiac) α-actinin antibody (clone EA-53; Sigma-Aldrich) and rhodamine-conjugated donkey anti-mouse IgG antibody (Jackson ImmunoResearch Laboratories). Cells were washed and antibodies were diluted (1/50) with PBS containing 1.5% horse serum. Micrographs of fluorescent cells (cells expressing immunomarked α-sarcomeric actinin and/or transgenic GFP) were taken with a Nikon Digital Sight DS-Qi1Mc camera mounted on a Nikon Eclipse Ti microscope equipped for epifluorescence. The fluorescence signals were analyzed with ImageJ software (National Institutes of Health) using the threshold function. The results were reported as percentages, comparing the fluorescent area with the total area on each micrograph. These analyses were done on unmerged red and green fluorescence micrographs, which were taken at 100× magnification and from culture fields characterized by cellular confluency. Therefore, the total area on each micrograph uniformly corresponded to the culture area occupied by cells. At least, three fields were considered per dish.
Immunoblotting
Cells were washed with PBS and harvested in an RIPA buffer (150mM NaCl, 50mM Tris, pH 7.6, 1% Nonidet P-40, 0.5% deoxycholate, and 0.1% sodium dodecylsulfate [SDS]) containing protease inhibitor cocktail I (Sigma-Aldrich) and the phosphatase inhibitors sodium orthovanadate (1mM) and sodium fluoride (1mM). Cell suspensions were stored at −20°C until analysis. On the day of analysis, cell suspensions of a given cell series were thawed, homogenized by up-and-down aspirations, and clarified by centrifugation to obtain the total cell lysates. The protein concentration of lysates was determined with a Micro BCA Protein Assay kit (Pierce), and aliquots (40μg) were loaded onto 10% polyacrylamide–SDS gels, electrophoresed for 60min at 150 V using a MiniProtean TetraCell system (BioRad), and electrotransferred onto polyvinylidene difluoride membranes for 30min, at 100 V, using the Criterion Blotter apparatus (Biorad). Membranes were blocked 60min in Tris buffer saline (TBS) containing 0.1% Tween-20 and 3% bovine serum albumin, and successively probed (60min each time) with a primary antibody (dilution 1/1000) and horseradish (HRP)-conjugated secondary antibody (dilution 1/10000). Antibodies were incubated and membranes were washed in TBS containing 0.1% Tween-20. Immune complexes were revealed by incubation of membranes with the Immobilon™ Western Chemiluminescent HRP substrate (Millipore) and exposition to HyBlot CL™ films (Denville Scientific). Films were analyzed by densitometry using ImageJ software (National Institutes of Health). Rabbit anti-octamer-binding transcription factor 3/4 (Oct3/4) antibody (H-134) and goat anti-cardiac troponin inhibitor (cTpnI) antibody (D-12) were from Santa Cruz Biotechnology. Rabbit anti-ERK1/2, anti-phospho-ERK1/2, anti-p38, and anti-phospho-p38 antibodies were from Cell Signaling Technology. Membranes were reprobed with mouse monoclonal anti-β-actin antibody (Sigma-Aldrich) or rabbit anti-α/β-tubulin antibody (Cell Signaling Technology) for normalization. Secondary antibodies, conjugated to HRP, were from Jackson ImmunoResearch Laboratories or Biosource International.
Statistics
JMP IN 8.0 Software (SAS Institute, Inc.) was used for statistical analysis. Means between cell populations were compared using Student's t-test (significance level P<0.05). Results were obtained from at least three independent cell series.
Results
Effect of retinoids on cell proliferation and stemness
The capacity of a substance to act as an inducer of stem cell differentiation is often associated with its capacity to reduce cell proliferation and to induce the loss of the cell stemness character. AtRA and the synthetic analogs used in this work were compared by these two criteria. As shown in Fig. 1B, the analogs were TTNPB, an RAR-specific agonist that recognizes all RAR isoforms (pan-RAR agonist); LG100815/RARatg, a pan-RAR antagonist; LG268, a pan-RXR agonist; and LG101208/RXRatg, a pan-RXR antagonist [44–46]. They were all used at 10−6 M throughout for two reasons. First, this concentration of atRA is effective in concurrently inducing adipogenesis and myogenesis in the P19 cell line [12]. Second, according to radiometric ligand-binding assays using preparations of receptors, atRA and analogs have similar Kd values, in the 10nM range, for their respective retinoid receptors (atRA and TTNPB: [46–48]; LG268: [44]; LG100815/RARatg and LG101208/RXRatg: personal communication from Pharmaceutical Ligands), and there was thus no need to adjust the concentrations to compensate for different affinities. Similar Kd values of 10nM characterize the binding of 9-cis-RA to RXR [48]. P19-MLC2v-GFP cells were used in this work because of our particular interest for cardiomyogenesis. These cells express the GFP gene under the transcriptional control of the MLC2v promoter that is characterized by its cardiac ventricle-specific expression [41]. Apparition of GFP fluorescence in differentiating cultures allows following the generation of CM cells in real time [41]. P19-MLC2v-GFP cells behave like P19 cells in our differentiation protocols.
Cell proliferation was evaluated in cultures of adherent monolayers of P19-MLC2v-GFP cells exposed to each retinoid during 2 days (Fig. 2A). AtRA decreased the proliferation of these cells by 70% compared to NI cells. The two retinoid agonists, LG268 and TTNPB, had a similar antiproliferative action, reducing the cell density by 50% and 70%, respectively. In contrast, RXRatg and RARatg had no influence on the proliferation of P19 cells. RARatg had also been reported to have no effect on the proliferation of breast carcinoma MCF-7 cells, even if used at 10μM [49].
RAR and RXR agonists reduce the proliferation and stemness character of P19-MLC2v-GFP cells. (A) Cell proliferation. Cell monolayers were treated for 48h with no inducer (NI) or with the indicated retinoid, and stained with crystal violet. Absorbance values are expressed as the means±SEM of three independent studies. (B) Cell stemness. Cells were subjected to the mesodermal protocol in the absence (NI) or presence of the indicated retinoid inducer from D2 to D3, and analyzed for Oct3/4 expression at D3. Cells were also collected at D0 (undifferentiated cells) and at D2 (before retinoid treatment) for comparison. An immunoblot specimen and the densitograms (means±SEM) obtained from three independent cell series. Oct3/4 signal was normalized to tubulin and reported relatively to the corresponding NI-D3 condition. The inducer treatment was significantly different from the NI-D3 condition (*), and the retinoid treatment was significantly different from the atRA reference treatment (#) (P<0.05).
The stemness character was evaluated through the protein expression of Oct3/4 transcription factor [50,51]. It is well established that uncommitted P19 and ES cells express this factor and lose it during differentiation, in particular under the action of atRA [52]. Oct3/4 expression was measured in D0, D2, and noninduced (NI) D3 cell aggregates to analyze the temporal effect of aggregation on this marker. The Oct3/4 level diminished with time during aggregation, reaching 50% of the initial value after 3 days (Fig. 2B). A 24-h exposure (D2 to D3) to atRA, LG268 or TTNPB importantly decreased Oct3/4 expression (respectively, by 89%, 77%, and 71% compared to NI cultures at D3). The decreasing effect of LG268 and TTNPB was more pronounced in the presence of atRA. Similar to their lack of effect on cell proliferation, the retinoid antagonists had no significant effect on the Oct3/4 level, compared to NI treatment. AtRA still preserved its reducing effect on Oct3/4 expression in the presence of antagonists. Because retinoid agonists appeared to stimulate cell differentiation by themselves while antagonists did not, we used two strategies to favor RAR and RXR signaling in turn: (i) stimulate RAR or RXR by replacing atRA by agonist TTNPB or LG268, and (ii) inhibit RXR or RAR by combining RXRatg or RARatg with atRA.
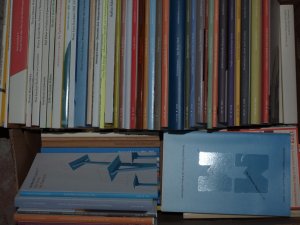
TTNPB is more potent than atRA in inducing adipogenesis
We and others have shown that atRA can induce the ES and EC cells to differentiate into adipocytes [12,53]. The induction of P19-MLC2v-GFP cells with LG268 or TTNPB as an atRA substitute generated cells containing lipid droplets stained by Oil-Red-O triglyceride dye (Fig. 3B–D). These fat cells were not found in corresponding NI cultures (Fig. 3A) and in undifferentiated cultures (D0, not shown). Quantification of staining showed that of the three retinoid agonists tested, TTNPB was the most potent inducer of triglyceride production in cultures (Fig. 3E, F). Gene expression of the transcription factor PPARγ and of the lipolytic enzyme LPL, another established adipocyte marker [54], was higher in induced than in NI cultures by qPCR analysis (Fig. 3G). Like triglyceride measurements, gene expression data underlined the important adipogenic action of TTNPB.
TTNPB is a potent adipogenic retinoid. Cell cultures were submitted to the mesodermal protocol and analyzed at D7+20. NI or the indicated retinoid inducer was used during the D2 to D5 period. Oil-Red-O staining micrographs (100×) of (A) NI, (B) atRA, (C) TTNPB, and (D) LG268 cultures. (E) Percentages of the culture surface occupied by Oil-Red-O-stained cells. (F) Histograms of Oil-Red-O absorbances standardized by crystal violet absorbances. Oil-Red-O absorbances were higher than expected in NI cultures by comparing with micrography results; this was due to the nonspecific trapping of the dye between cells in these highly proliferating cultures. (G) RT-qPCR analysis of LPL and PPARγ, respectively, normalized to β-actin and GAPDH signals. All results (means±SEM) are for three independent cell series. The inducer treatment was significantly different from the NI condition (*), and the retinoid treatment was significantly different from the atRA reference treatment (#) (P<0.05). Color images available online at www.liebertpub.com/scd
LG268, but not TTNPB, induces myogenesis
We previously showed that skeletal and cardiac myogenesis accompanies adipogenesis in P19 cells treated with atRA [12]. The muscle cells appear as soon as 10 days postaggregation (D7+10) [12]. In this work, we used cytofluorescence to determine whether replacing atRA by synthetic RAR- and RXR-selective agonists would have an impact on myogenesis. Cells exhibiting both red fluorescence (immunologically α-actininPOS) and green fluorescence (GFPPOS) on unmerged micrographs, or green or yellow fluorescence (GFPPOS and α-actininPOS) on merged micrographs, were CM, whereas cells only exhibiting red fluorescence (α-actininPOS) were mainly SKM. The CM and SKM phenotypes could be confirmed by morphology, CM having a rounded appearance and SKM an elongated shape. atRA and LG268 treatments generated SKM and CM cells with similar efficiencies (Fig. 4B, D, E). Similar to NI cultures (Fig. 4A), there was no brilliant green or red fluorescence in TTNPB-treated cultures (Fig. 4C, E). TTNPB has thus an antimyogenic influence. Like cytofluorescence data, Western blots of sarcomeric α-actinin and cTpnI indicated lower expression of these myomarkers in cultures treated with TTNPB than in those treated with atRA and LG268 (Fig. 4F). Since cTpnI is a general marker of CM cells [55,56], the blotting results showed a tendency of LG268 to generate a higher proportion of muscle cells of the cardiac type compared to atRA.
Unlike atRA and LG268, TTNPB is antimyogenic. Cell cultures were submitted to the mesodermal protocol until D7+10 and analyzed for GFP and immunoreactive myomarker expression. Fluorescence micrographs (100× or as indicated) of (A) NI cultures and cultures induced with (B) atRA, (C) TTNPB, and (D) LG268. Photonic micrographs show the cell culture densities. (E) Relative fluorescence histograms (means±SEM of three independent cell series analyzed in triplicate). (F) Immunoblot specimens and densitograms (means±SEM of three independent cell series). The α-actinin and cTpnI signals were normalized to actin and reported relatively to the corresponding atRA condition. The retinoid treatment was significantly different from the atRA reference treatment (#) (P<0.05). Color images available online at www.liebertpub.com/scd
There are two apparent discrepancies between cytofluorescence and immunoblotting results. First, there was basal expression of myogenic markers in TTNPB cultures by immunoblotting (Fig. 4F) despite the absence of corresponding cytofluorescence signals (Fig. 4C, E). A part of the immunoblotting signals could have come from the presence of abundant precursor cells expressing basal levels of myogenic markers in the cultures. Indeed, it has been shown that markers of various mature cell types can be expressed altogether, at basal levels, in multipotent precursor cells, and in the process of cell type specification, the specific markers of a given cell type are upregulated, while the other ones gradually disappear [57]. The second discrepancy concerns signal intensities. Indeed, cytofluorescence data showed strong differences in myomarker expression between TTNPB cultures and cultures induced with atRA or LG268, whereas these differences were modest by immunoblotting. This could be explained by the rarity of muscle cells in the cultures relatively to cells expressing basal levels of markers. Consequently, even if muscle cells exhibited strong cytofluorescence signals, these signals would have been diluted by the contribution of week signals coming from many other cells. In cases of low differentiation yields, cytofluorescence, compared to immunoblotting, provides a better appraisal of the proportion of cells that differentiate along a particular pathway in cultures.
Timecourse of ERK and p38 phosphorylation in cultures treated with atRA and retinoid agonists
Several studies have analyzed the activity of ERK and p38 in ES cells subjected to mesodermal differentiation [23,24,33]. However, to our knowledge, no study has examined the impact of retinoids on these MAPK during this process. In this work, we observed a transient increase in ERK phosphorylation when cells began to form embryoid bodies (D0–D2, all cultures) (Fig. 5A–D). In NI cultures, this initial increase was followed by a decrease to below the basal levels until D6, and then a return to initial values and above until D9, which was the last day analyzed. The introduction of retinoid agonists at D2 had an impact. AtRA exalted the reduction in ERK phosphorylation in the D3-to-D6 period compared to the NI condition (Fig. 5A, B). The effect of LG268 on ERK resembled that of atRA (Fig. 5C), whereas the effect of TTNPB was slightly more potent (Fig. 5D). Like for ERK, p38 phosphorylation increased from D0 to D2 during the formation of embryoid bodies (Fig. 5E–H). Thereafter, in NI cultures, p38 phosphorylation almost plateaued until D6 instead of decreasing like ERK phosphorylation (Fig. 5F). RA and LG268 treatments similarly stimulated p38 phosphorylation over NI levels, from D4 (Fig. 5F, G). In contrast, p38 phosphorylation levels in TTNPB-induced cultures matched those of NI cultures (Fig. 5H). Overall, atRA, LG268, and TTNPB treatments had similar effects on ERK phosphorylation, but, contrary to atRA and LG268, TTNPB had no phosphorylating effect on p38.
Time course of ERK and p38 phosphorylation in differentiating cultures. NI cultures and cultures induced with atRA, TTNPB, and LG268 were analyzed for phosphorylated (p) and total ERK and p38, during the differentiation induction period (D0 to D7) and at D9. (A, E) Immunoblot specimens. (B-D, F-H) Comparative densitograms (means±SEM of three independent cell series). The results of each series (p-ERK/total ERK and p-p38/total p38) are reported relatively to the corresponding D2. The actin signals (not shown) followed those of total ERK and p38. Notice, in H, the comparison of TTNPB with NI instead of with atRA.
Effects of the combination of atRA and MAPK signaling inhibitors on mesodermal differentiation
We next wanted to determine whether interfering with ERK or p38 signaling during atRA treatment could influence mesodermal differentiation. PD98059, which inhibits MEK-mediated ERK phosphorylation, was used to interfere with ERK signaling, and SB203580, which inhibits the activity of phospho-p38, was used to interfere with p38 signaling. On the one hand, we believed that addition of PD98059 to sustain the low levels of phospho-ERK already observed during the induction phase (Fig. 5B–D) would increase myogenesis and perhaps decrease adipogenesis, since ERK signaling was reported to be important in the generation of adipocytes [33]. On the other hand, considering that the stronger adipogenic action of TTNPB compared to atRA was associated with lower p38 phosphorylation, we reasoned that addition of SB203580 could enhance the adipogenic action of atRA, but not its myogenic influence. As shown in Fig. 6, PD98059 (10μM) enhanced the myogenic efficiency of atRA (panels G, H, J), but not its adipogenic influence, which remained unchanged (panels A-E). SB203580 (10μM) stimulated, by about two-fold, the adipogenic action of atRA, and this effect was observed as soon as D7+14. Like for the ERK inhibitor, α-actinin fluorescence data showed that SB203580 doubled the myogenic action of atRA (Fig. 6F–J). According to the MLC-2v-GFP fluorescence, the ERK inhibitor even appeared to stimulate the cardiomyogenic action of atRA compared to the p38 inhibitor.
The combinations of atRA with inhibitors of ERK and p38 signaling are adipogenic and myogenic. Cells were induced with atRA alone or in combination with PD98059 (PD) or SB203580 (SB) from D2 to D5, and analyzed for myogenesis at D7+10 and for adipogenesis at D7+14. Oil-Red-O staining micrographs (100×) of (A) NI cultures and cultures induced with (B) atRA, (C) atRA+PD, and (D) atRA+SB. (E) Percentages of the culture surface occupied by Oil-Red-O-stained cells. (F-I) Photonic and myomarker fluorescence micrographs (100×). (J) Relative fluorescence histograms. All results (means±SEM) are for three independent cell series analyzed at least in triplicate. Significant difference from the atRA reference treatment (#) (P<0.05). Color images available online at www.liebertpub.com/scd
Effects of the combination of retinoid antagonists and MAPK-signaling inhibitors on mesodermal differentiation
Finally, we studied the effects on mesodermal differentiation of inhibiting RXR and RAR signaling in turn while stimulating cell differentiation with atRA. Combining atRA with RXRatg or with RARatg was as adipogenic as atRA itself (Fig. 7A and Supplementary Fig. S1). The combination atRA+RARatg was as myogenic as atRA, while that of atRA+RXRatg gave lower muscular yields (Fig. 7B and Supplementary Fig S2). The addition of ERK inhibitor had no effect on mesodermal yields of atRA+RXRatg and atRA+RARatg treatments (Fig. 7 and Supplementary Figs. S1 and S2). However, the p38 inhibitor amplified the partial antimyogenic effect of atRA+RXRatg treatment and blocked the adipogenic and myogenic effects of atRA+RARatg treatments (Fig. 7 and Supplementary Figs. S1 and S2). These severe inhibitory impacts on adipogenic and myogenic yields were not due to a general inhibition of cell differentiation, since all combinations of atRA with retinoid antagonists and MAPK inhibitors revealed to reduce cell proliferation and Oct3/4 expression similar to atRA itself (Supplementary Fig. S3).
Oil-Red-O staining (A) and myomarker expression (B) in cultures treated with combinations of atRA, RAR/RXRatg, and MAPK inhibitors. Analyses were done as indicated in the legend to Figures 3, ,4,4, and and6.6. Results (means±SEM) are for three independent cell series, and the symbols # and * indicate a significant difference from atRA treatment and from corresponding treatment without MAPK inhibitor, respectively (P<0.05). Micrograph specimens are shown in figures 1S (Oil-Red-O) and 2S (myomarkers).
Discussion
P19 stem cells can differentiate into various cell lineages in response to atRA. In particular, they give rise to adipocytes and to muscle cells of the cardiac and skeletal types, present altogether in the same culture, upon exposure to 10−6 M atRA during embryoid body formation. P19 cells thus provide a useful model to investigate the impact of modulating atRA signaling on mesodermal differentiation, with possible applications in the field of cell-based therapies. This work shows that atRA analogs can influence the relative yield of adipocytes and muscle cells depending upon their specificity toward RAR and RXR. In addition, atRA and agonist analogs have their own influence on the phosphorylation of ERK and p38, which are two MAPK implicated in stem cell differentiation. Although the exact mechanisms relating the RAR/RXR ligands and MAPK in this work are not known, the results raise the possibility of selecting atRA analogs according to their effect on MAPK phosphorylation in addition to their capacity to act on RAR/RXR, for the purpose of cell differentiation. atRA analogs with morphogenic potential could be rapidly identified through their effects on MAPK, since these effects occur before expression of markers of mature cells during cell differentiation. Finally, pharmacological inhibition of ERK and p38 activity can further modulate retinoid actions. Table 2S summarizes the effects of retinoids and MAPK inhibition on mesodermal yields, comparing with atRA treatment, and Fig. 8 schematizes the main findings of this study.
Some aspects of the RAR/RXR and MAPK relationships in mesodermal differentiation of P19 cells. Lines ending with an arrowhead indicate stimulatory effects, and lines ending with a small perpendicular bar indicate inhibitory effects. Activation of RAR and RXR by atRA concurrently induces adipogenesis and myogenesis. Treatments favoring RAR over RXR signaling preferentially induce adipogenesis over myogenesis (TTNPB and atRA+RXRatg treatments). Treatments favoring RXR over RAR signaling permit both differentiation routes (LG268 and atRA+RARatg treatments). Inhibitors of p38 (p38i) and ERK (ERKi) signaling have differential effects on mesodermal differentiation depending upon the cellular retinoid status. P38i enhances myogenesis and adipogenesis when RAR and RXR are activated, inhibits both differentiation routes under the predominance of RXR signaling, and increases the antimyogenic action of RAR when RAR signaling predominates. ERKi increases myogenesis when RAR and RXR are activated, but has no influence on myogenesis or on adipogenesis when either RAR or RXR signaling predominates.
This study shows that favoring RAR activity over RXR activity has proadipogenic and antimyogenic impacts (Fig. 8). RAR activity is proadipogenic as illustrated with the use of TTNPB to preferentially activate RAR (Fig. 3 and Supplementary Table S2: treatment 3) and with the use of atRA in conjunction with RXRatg to preferentially deactivate RXR (Fig. 7 and Supplementary Table S2: treatment 4). The critical role of RAR in adipogenesis was revealed by comparing atRA and atRA+RARatg treatments when p38 signaling was inhibited (Fig. 7 and Supplementary Table S2: treatments 10 and 12). Indeed, in the presence of the p38 inhibitor, RARatg abolished atRA-induced adipogenesis in P19 cells. This is in accordance with the work of Monteiro et al., showing the inhibitory action of another RAR antagonist on atRA-induced adipogenesis in an ES cell line [15]. However, in that work in contrast to ours, the demonstration was not conditional to the inhibition of p38. For the first time is revealed a concurrent antimyogenic action of RAR signaling and this, in either absence or presence of p38 inhibitor (Fig. 8). Indeed, in both p38 situations, the SKM+CM and CM yields were null or reduced with the use of TTNPB or atRA+RXRatg compared to the corresponding atRA treatment (Figs 4 and and77 and Supplementary Table S2: treatments 3 and 4 versus 2, and treatment 11 versus 10). The antimyogenic effect of TTNPB was greater than that of atRA+RXRatg, which could be due to the stability of TTNPB in cell culture. TTNPB was indeed reported to be more stable than atRA, which led to a more prolonged stimulatory action on RAR compared to atRA [58].
Favoring RXR over RAR activation in the absence of p38 inhibitor induced myogenesis (Fig. 8). Indeed, LG268 and atRA+RARatg were as myogenic as atRA itself (Figs 4, ,77 and Supplementary Table S2: treatments 5 and 6 versus 2). A myogenic influence of RXR activation has been reported in two other studies. In one study, an RXR agonist was shown to stimulate, and an RXR antagonist to inhibit, the spontaneous cardiomyogenic differentiation of ES cells [13]. In the other study, treatment with an RXR agonist induced skeletomyogenesis in ES cell cultures and stimulated the skeletomyogenic action of dimethyl sulfoxide (DMSO) in P19 cell cultures [14]. In our cell system, the antimyogenic impact of RXRatg in association with atRA can be due to RXR inactivation, but also to RAR activity supplanting RXR activity. In contrast to RAR, which directs cells toward adipogenesis preferentially to myogenesis, RXR permits adipogenesis in addition to myogenesis.
Several studies have implicated p38 and ERK in mesodermal differentiation of stem cells. p38 has been proposed to act as a negative regulator of adipogenesis, since pharmacological or genetic downregulation of p38 signaling increased the adipogenic yield in atRA-induced cultures of ES cells [21]. We obtained similar results with P19 cells, since atRA+p38 inhibition yielded more adipocytes than atRA alone (Fig. 6 and Supplementary Table S2: treatment 10 versus 2). However, p38 inhibited instead of enhanced adipogenesis in the presence of RARatg (Fig. 6 and Supplementary Table S2: treatment 12 versus 6). This would indicate that the retinoid status of the cell is influential (Fig. 8). Inhibition of p38 was reported to be antimyogenic. Indeed, pharmacological inactivation of p38 inhibited cardiomyogenesis in DMSO-induced P19 cultures [24], and p38 knockout in ES cells reduced the spontaneous generation of SKM and CM [17]. It is worth noting that in those cases compared to our study, myogenesis was triggered in the absence of exogenous atRA or retinoid. In addition, the impact of the p38 inhibitor SB203580 on cardiomyogenesis was found to be concentration dependent, stimulating at ≤10μM and inhibiting at higher concentrations [27–29]. Because of our desire to increase the myogenic yields, we used the inhibitor at 10μM with atRA and found equivalent and even ameliorated yields compared to atRA alone (Fig. 6 and Supplementary Table S2: treatments 7 and 2). In contrast, p38 inhibitor, at the same concentration, had an inhibitory influence on myogenesis when either RAR or RXR were inactivated (Fig. 7 and Supplementary Table S2: treatments 11 and 12 versus 10, 4, and 6). Inhibition of ERK signaling was shown to have a little effect in cardiac differentiation of P19 cells induced with DMSO [23,24] or in the spontaneous cardiomyogenesis of ES cells [33]. We observed increased myogenic yields (Fig. 6 and Supplementary Table S2: treatments 7 and 2) by inhibiting ERK signaling in our atRA-mediated mesodermal differentiation protocol. This myogenic enhancement vanished by incorporating RXRatg or RARatg in these experiments, which again points to the influence of the cellular retinoid status, as it was the case with p38 inhibitor.
Overall, our results showing the importance of RAR in adipogenesis and of RXR in myogenesis are in consistence with literature, while this is not always the case for MAPK data. It appears that changes in the cellular retinoid status (including variations in the levels of retinoids or of RAR/RXR expression/activity) are influential (Fig. 8). This is well illustrated with the use of p38 inhibitor, since the same concentration of p38 inhibitor can stimulate or inhibit a given mesodermal route depending upon the retinoid combination used to induce differentiation. This aspect of retinoid status could explain some inconsistencies observed between in vitro and in vivo situations. For instance, while some studies have implicated p38 as an important player in cardiomyogenesis in cell cultures, various strategies of p38 knockout resulted in normal development of the heart [17,20]. In the same line of thought, it is worth of note that retinoid agonists had different effects on differentiation into visceral endoderm in cell cultures depending upon which RAR and RXR had been knockout [59].
Another aspect to consider while comparing the results from different treatments is the possibility of noncell autonomous effects. In this work, as well as in all other studies of mesodermal differentiation of stem cell cultures, muscle cells and adipocytes represent a small proportion of cells. Treatments that abolish generation of these cells may not inhibit all differentiation. This seems to be the case in our work as suggested by downregulation of cell proliferation and Oct3/4 expression (Supplementary Fig. S3). Cells that remain in their undifferentiated state as well as cells that take other differentiation routes could influence the environment of myogenic and adipogenic precursor cells, through either cellular contacts or secretion of factors. The spectrum as well as the metabolic status of conditioner cells could change from one stem cell system to another or in response to variations in differentiation protocols. Consequently, these changes could modify the influence of these cells on the generation of the cell type of interest. In vitro and/or in vivo, the development of CM cells can benefit from noncell autonomous effects originating from the endoderm, pericardium, and endocardium [28,60,61], and the development of adipocytes from influence of the developing vasculature and neural crest [62].
In conclusion, the strategy of combining retinoids and pharmacological modulators of MAPK signaling can help in directing cell differentiation in culture, but combinations may have different influences depending upon the stem cell system used and its retinoid status. In this work, this strategy has succeeded in improving adipogenesis and isolating it from myogenesis, but myogenesis, even improved, was accompanied by adipogenesis, and antiadipogenic conditions were also antimyogenic.
Supplementary Material
Acknowledgments
This work was supported by a grant from the Canadian Institutes of Health Research. Studentships afforded to F.B. by the UQAM's FARE program and Pharmaqam Research Center are gratefully acknowledged. We are also indebted to Dr. Christine L. Mummery (Department of Anatomy and Embryology, Leiden University Medical Centre, Leiden, The Netherlands) for the generous gift of P19CL6-MLC2v-GFP cells, to Dr. Jennifer C. Moore for useful information on the culture of these cells, and to Dr. Reid P. Bissonnette (Ligand Pharmaceuticals, Inc.) for graciously providing the LG100268, LG100815, and LG101208 ligands.
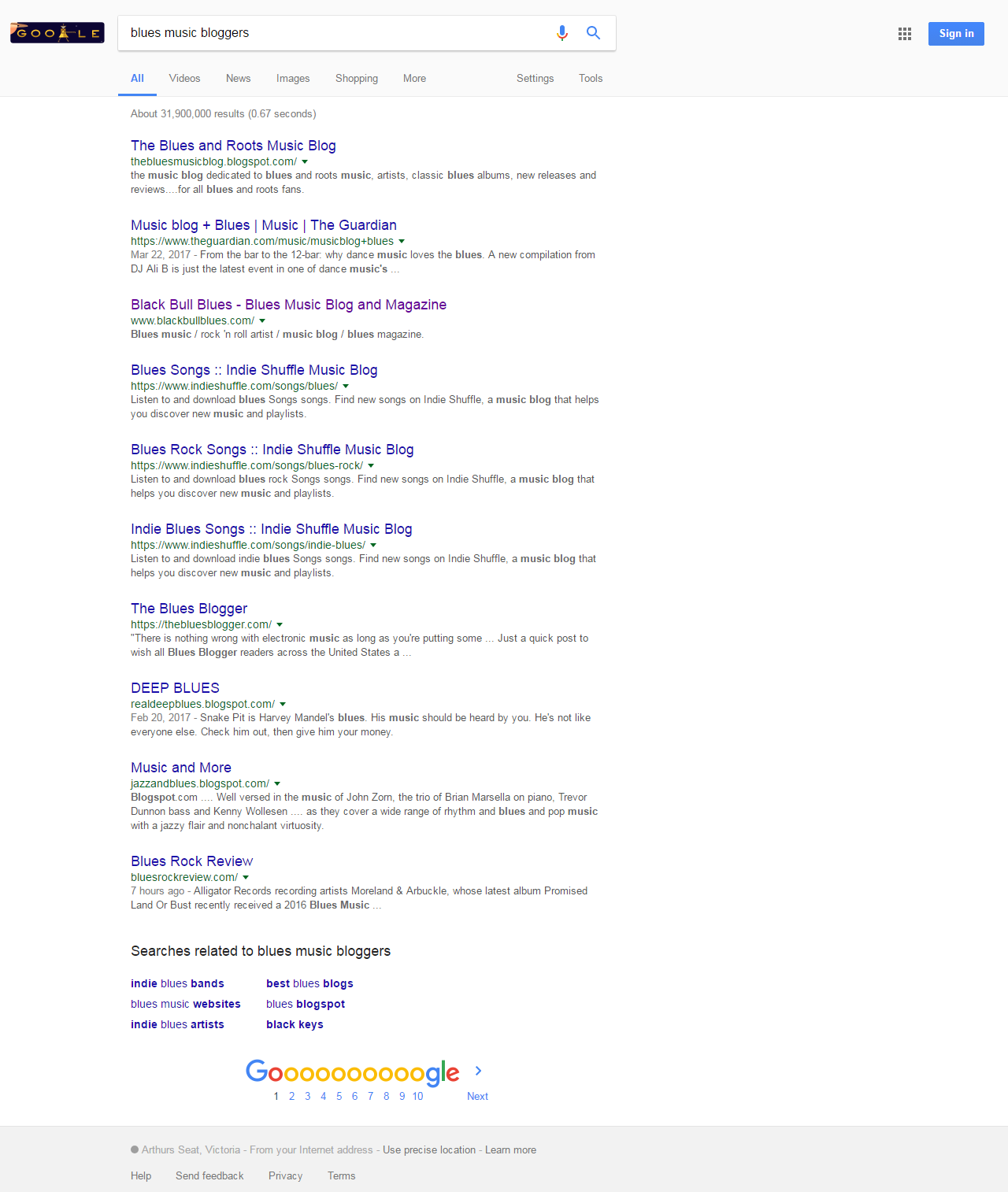
Author Disclosure Statement
The authors declare no conflict of interest.
References
Complete Single Collection
